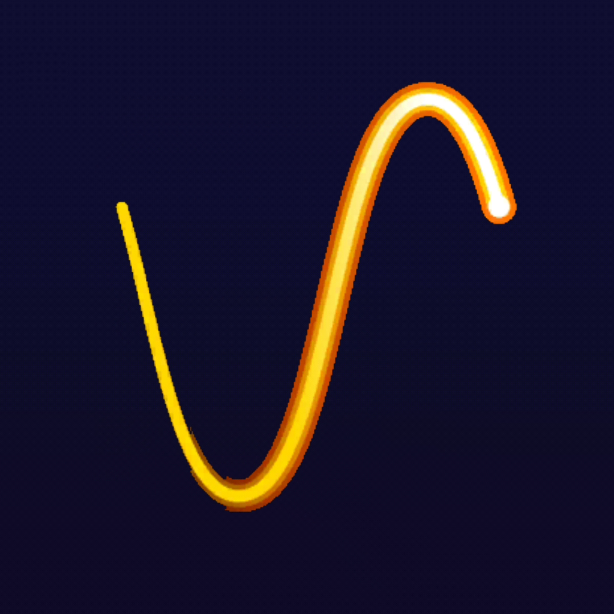
Don’t mess with gamma rays. Gamma ray photons, with their super short wavelength, are more energetic than a million visible photons combined. Energetic enough to damage cells, they can wreak havoc on a human body. Luckily for us, our atmosphere’s ozone layer filters out gamma rays. Certain black holes can emit “gamma ray bursts”, two ridiculously powerful, tightly-focused jets of gamma rays that unleash more energy in a second than our Sun will produce in its entire lifetime. If one of these happened anywhere within a few thousand light years of us, we would be toast.