For centuries, physicists all over the world were leading a heated debate about the nature of light and for many years there were two opinion groups among them. Supporters of the first group believed that light was a wave, while members of the other group believed that electromagnetic radiation had a particle nature. However, quantum mechanics showed that neither of the groups was completely right and that the real answer to this question is much stranger and much more complicated than any of the contemporary thinkers could have ever imagined.
Young’s Experiment
Young’s experiment, often referred to as the double-slit experiment, is a relatively simple experiment. It was used at the beginning of the 19th century to prove that light exhibits wave properties. This experiment exploits two specific properties of waves:
- If a wave reaches a small opening, it bends. This phenomenon is called diffraction. The size of the opening has to be comparable to the wave’s wavelength for diffraction to occur.
- When two waves encounter, they do not collide but strengthen or weaken each other depending on what the displacement (“height”) of both waves is. This phenomenon is called interference. For example, when two waves with opposite displacements meet (i.e. a crest of one wave meets a trough of another wave), they cancel out. If interfering waves weaken each other, we are talking about destructive interference. The opposite of destructive interference is constructive interference (waves strengthen each other).
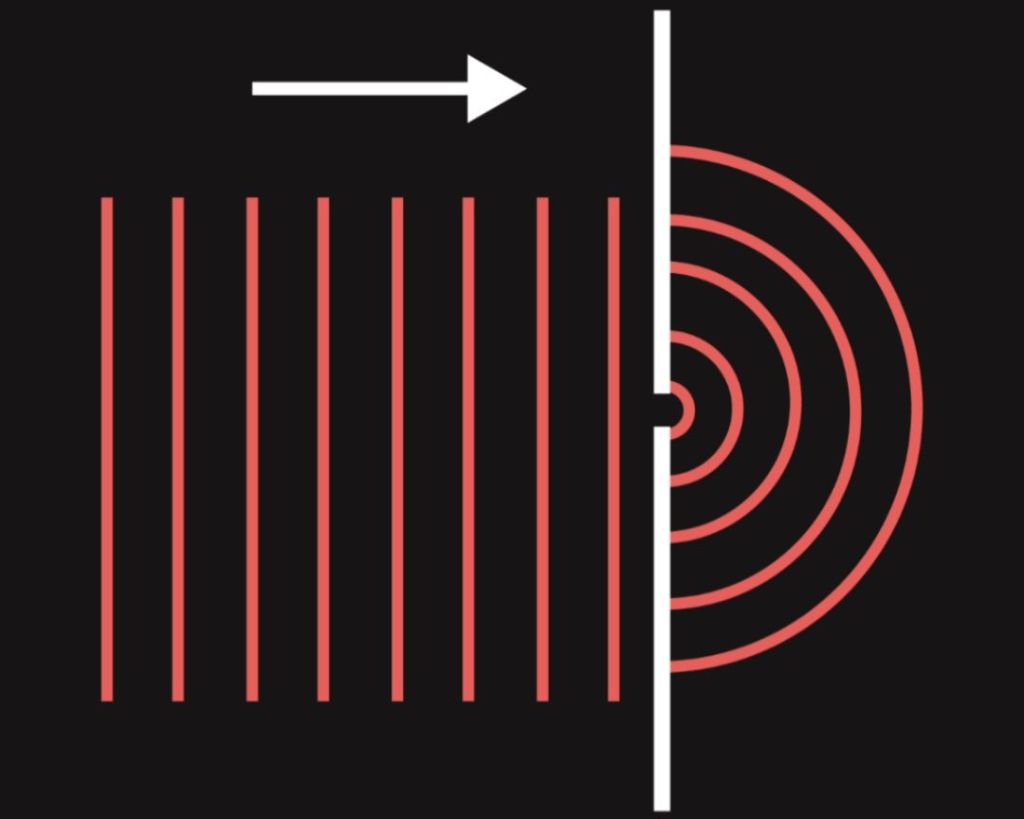
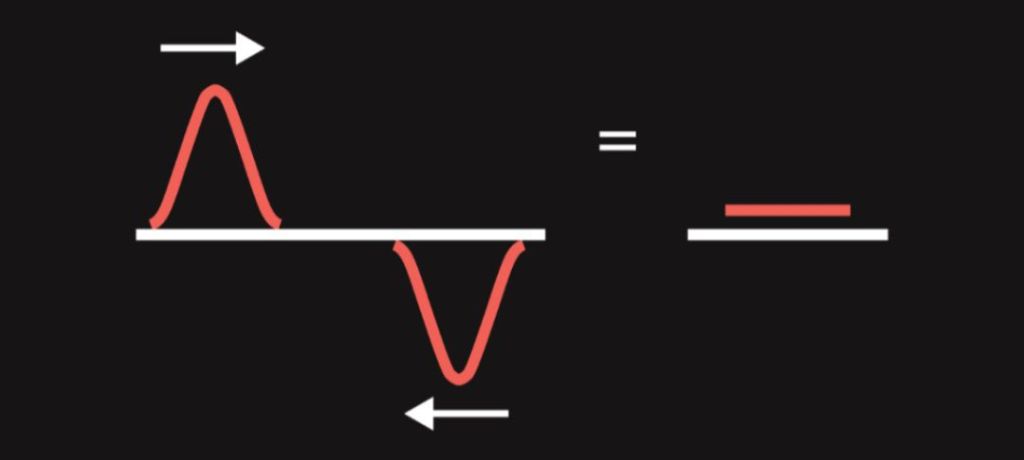
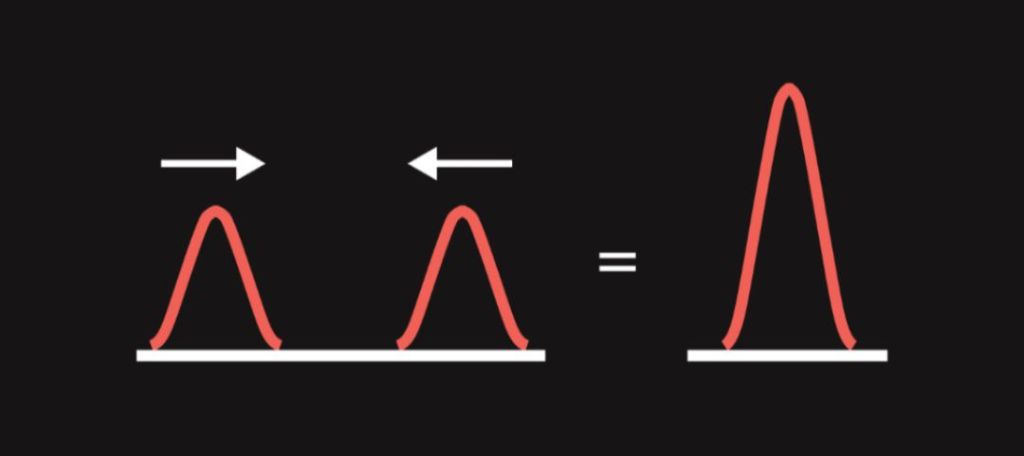
In the double-slit experiment, two slits, which are very close to each other, are used. Light passes through both slits and spreads to the medium behind the opening thanks to diffraction. Due to small distance between the slits, the waves from the first slit meet the waves from the second slit and interference occurs. If we situate a plate detecting the position of individual beams of light that strike it, a specific pattern is created, the so-called interference pattern, which consists of light and dark stripes. Light stripes on the plate are located in places where constructive interference of light waves occurs (waves strengthen one another, thereby increasing the intensity of light incident on these places), dark stripes are caused by destructive interference (waves weaken one another, thereby decreasing the light intensity). If light did not exhibit wave properties, interference pattern would not be created.
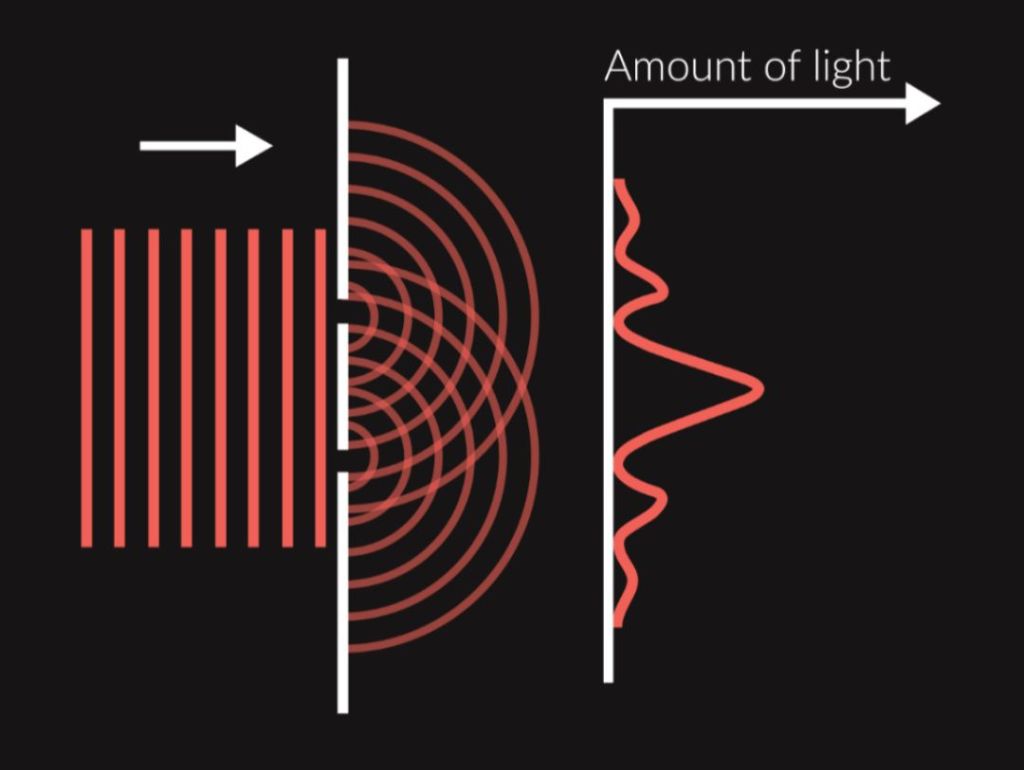
Young’s experiment is a simple experiment demonstrating the wave nature of electromagnetic radiation. The original version of this experiment is not related to quantum mechanics, but using its modifications, we can easily prove some of the strange phenomena of the microworld, as we shall see in the following chapters.
Photoelectric Effect
According to the Bohr model of the atom, it is necessary to provide electrons with energy in the form of electromagnetic radiation in order for them to get to orbitals with higher energy. However, if an electron absorbs a wave of high frequency (and thus energy), sometimes this energy is sufficient for the electron to abandon the atom entirely. This phenomenon, when electrons are released from the shell of an atom, is called the photoelectric effect. Electrons that are released in this manner are called photoelectrons.
Let us imagine an experiment where one shines light on electrons inside of an atom, whereby some of these electrons are released from the atomic shell and become photoelectrons. Classical physics states that the energy of photoelectrons should be dependent on the intensity of light, since it assumes that the higher the intensity of radiation (i.e. the brighter the light is), the higher the energy of the electromagnetic wave that is then absorbed by the electrons. Nevertheless, this dependency has not been observed. It was experimentally proven that the energy of the emitted electrons depends purely on the frequency of radiation. Also, the existence of the so-called threshold frequency has been observed. If one provides an atom with light that has lower frequency than the threshold frequency, no electrons are released, again regardless of the intensity of radiation. Classical physics is not able to explain this phenomenon.
Young’s experiment presents a very convincing evidence that light is a wave. However, in order to explain the photoelectric effect, we need to perceive light as a set of particles. Electrons do not absorb electromagnetic waves as predicted by classical mechanics. They absorb particles of light, called photons. Photons are identical to the energy quanta Planck proposed to solve the problem with black-body radiation. Einstein, however, was the first one to realize the particle nature of these quanta, and it was him who managed to clarify the photoelectric effect.
If we perceive light as a stream of particles, the photoelectric effect can be explained quite easily. Increase in intensity of radiation raises the number of photons (quanta) in an electromagnetic wave, but individual photons still carry the same amount of energy. That is to say, if one uses more intense light, the energy of photoelectrons stays unchanged, since electrons may absorb only one photon, in accordance with the third Bohr’s rule. However, by increasing the intensity of light, the number of emitted electrons (photoelectrons) is increased as there are now more photons in electromagnetic waves to be absorbed.
If we wanted to raise the energy of photoelectrons, we would have to raise the energy of individual photons. We could achieve that by raising the frequency of radiation, which is obvious from Planck’s equation E = h ⋅ f (E is the energy of a photon). Quantum mechanics is also able to explain the threshold frequency. Individual photons of low-frequency radiation simply do not have enough energy to release an electron. Therefore, the photoelectric effect does not occur.
Albert Einstein also derived the equation for calculating the momentum of a photon (λ is the wavelength of the electromagnetic wave in which the photon is located):
𝐩 = 𝐡 / 𝛌
When an electron absorbs a photon, it obtains all of its energy. Part of this energy is then used to extricate the electron from the atom (the electron must accomplish work W), the rest of the energy is converted into the kinetic energy of the electron. The energy of a photoelectron can thus be calculated using the following equation:
𝐄 = 𝐖 + ½ 𝐦𝐯𝟐
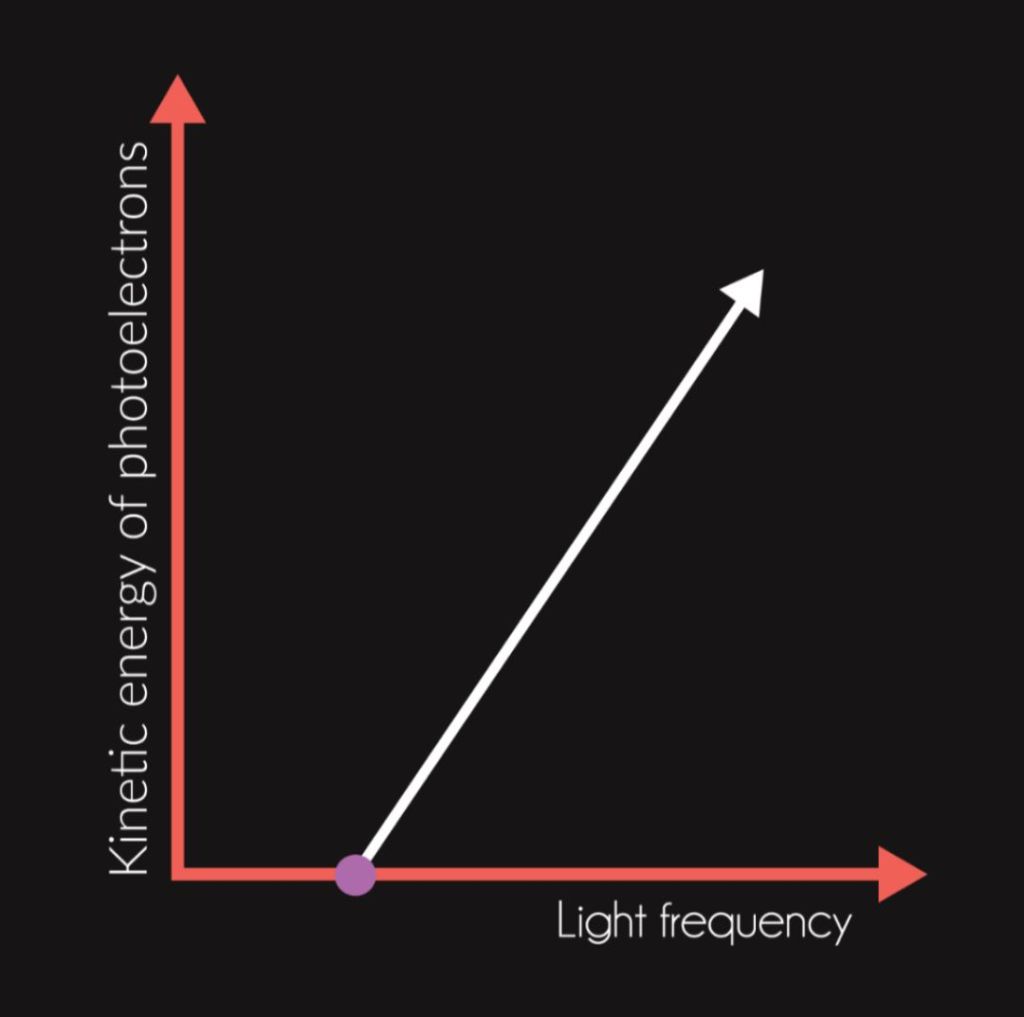
While Young’s experiment convincingly demonstrates the wave nature of light, the photoelectric effect sees light as a stream of particles. Therefore, electromagnetic radiation is dual in nature. It has both wave and particle nature.
Matter Wave
After a partial clarification of the strange properties of light in the form of the wave-particle duality comes the year 1924 and a young French physicist Louis de Broglie with a very daring hypothesis. According to this hypothesis, duality does not apply only to light but to every single object in the universe. In other words, de Broglie presumed that all objects, including the ones with mass, are surrounded by a kind of wave, similarly to photons. It is not a great surprise that this revolutionary hypothesis was initially not received very well. Opponents of the hypothesis argued that, after all, matter behaves nothing like a wave.
Eventually, however, it turned out that de Broglie had been right and that the so-called matter wave indeed exists. The presence of this wave can be demonstrated with the help of Young’s experiment, for instance. When conducting the double-slit experiment with massive particles (electrons, for example), an interference pattern is created, which confirms de Broglie’s hypothesis. The relation between the momentum of an object and the wavelength of its matter wave is expressed by the following equation:
𝛌 = 𝐡 / 𝐩
The equation above shows that the wavelength of an object’s matter wave decreases when the momentum of the object increases. In other words, the more massive the object, the smaller the wavelength of its matter wave. This is why objects from the macroworld do not exhibit wave-like properties. Matter waves of large objects have very small wavelengths, which means that if we wanted to prove the wave nature of a large object using Young’s experiment, for example, we would encounter a problem. For diffraction and interference to occur, the size of the slits and the distance between them would have to be significantly smaller than the size of the object itself.
In quantum mechanics, light and matter are dual. Sometimes their wave nature comes to light, other times they show their particle nature. This ground-breaking idea is fundamental to quantum mechanics.